Hexaquarks as Dark Matter? The Physics Behind the Idea Explored
Written on
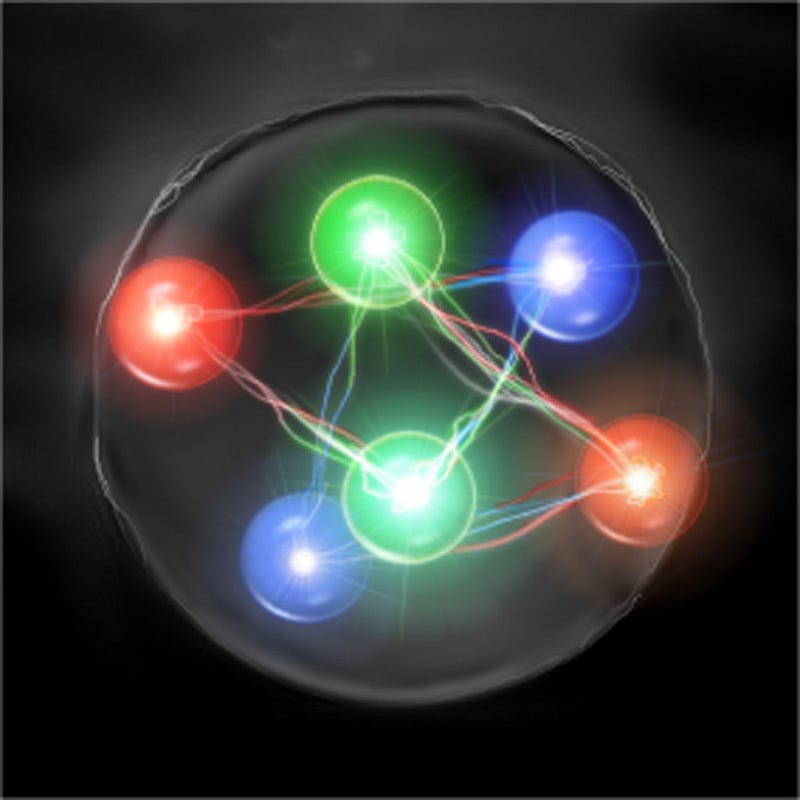
Ask Ethan: Is it Outlandish to Suggest Dark Matter Comprises Hexaquarks?
The existence of dark matter is a well-established scientific principle that helps account for various cosmic observations. While we understand its necessity, the exact particles that constitute dark matter remain elusive. Despite extensive research and numerous experiments aimed at direct detection, we've found no definitive evidence of any proposed dark matter candidates. Recently, a novel suggestion has emerged: the notion that hexaquarks might serve as viable dark matter constituents. Patreon supporter BenHead poses the question:
> Many headlines indicate dark matter could be a Bose-Einstein condensate of d hexaquarks. However, I notice that detected d* hexaquarks had a lifespan of just 10^-23 seconds. What are your thoughts?*
This proposition is indeed intriguing but likely misguided for several reasons.
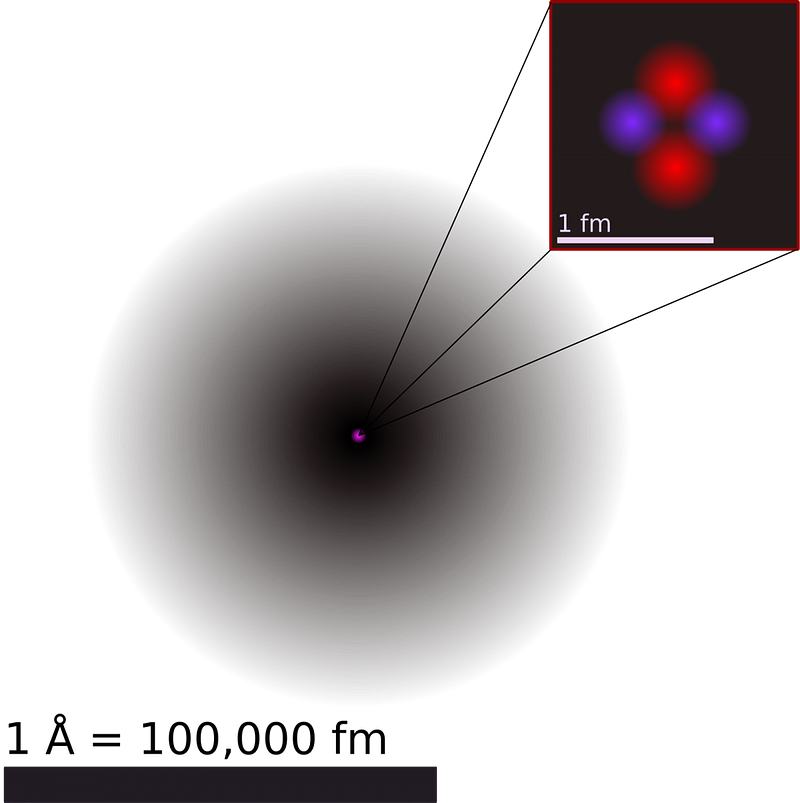
As we began to explore atomic nuclei, we discovered various intriguing properties:
- Atomic nuclei consist of protons and neutrons.
- Neutrons are marginally heavier than protons by about 0.1%.
- Free protons are indefinitely stable.
- Free neutrons have a mean lifetime of approximately 15 minutes before decaying.
- When protons and neutrons are bound together, the resultant nucleus has less mass than the sum of its parts.
- Certain combinations of these nucleons yield stable nuclei, while others lead to decay.
One decay mode, known as beta decay, involves a neutron transforming into a proton, an electron, and an anti-electron neutrino.
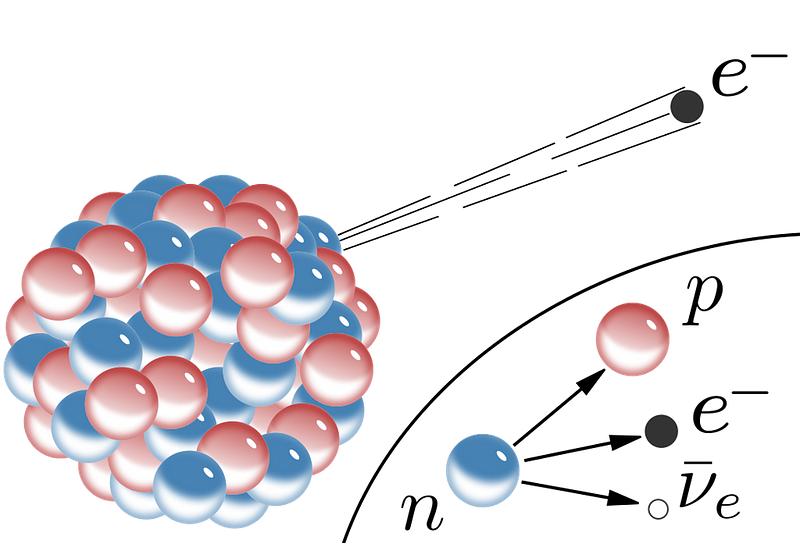
A crucial insight is that some unstable particles, when bound, can achieve stability. Free neutrons may decay, but neutrons within stable nuclei, such as those from helium to iron, remain stable indefinitely.
The reason for this stability lies in the binding energy relative to the mass-energy difference between the initial particle (neutron) and the decay products (proton, electron, and neutrino). A tightly bound system can result in stability even with inherently unstable components. Neutron stars exemplify this; despite being composed of neutrons, their gravitational and nuclear forces maintain overall stability.
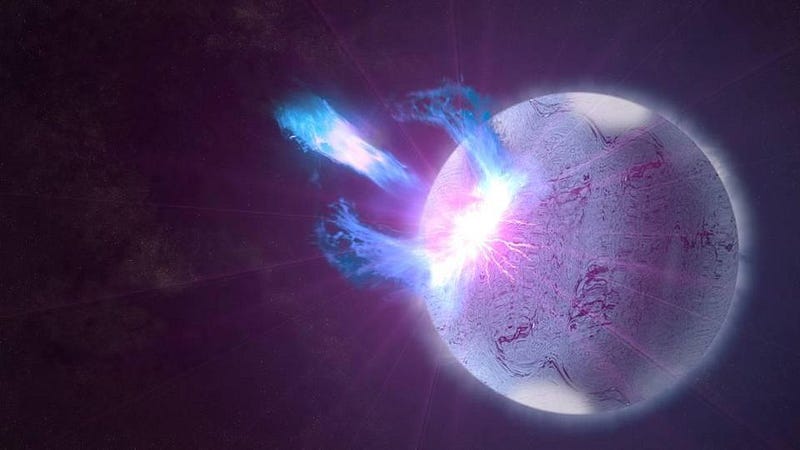
With a grasp on binding energy, physicists proposed explanations for the array of particles emerging from colliders. Among these findings were heavier, unstable particles such as the Lambda particle (??), along with various mesons and baryons.
In 1956, Shoichi Sakata suggested that these new particles might be composites of three fundamental particles:
- Proton
- Neutron
- ??
Although the Sakata model was later disproven by experiments confirming the existence of quarks and gluons, the idea persisted that unstable composites might achieve stability under certain conditions.
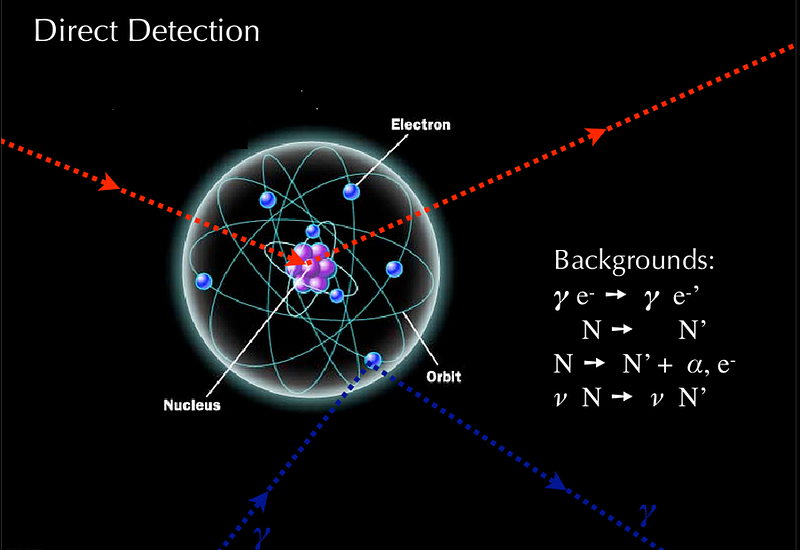
Today, we recognize various particles that can form stable configurations, such as:
- Baryons (three quarks each)
- Anti-baryons (three antiquarks)
- Mesons (quark-antiquark pairs)
- Tetraquarks (two quarks and two antiquarks)
- Pentaquarks (four quarks and one antiquark)
- Hexaquarks (six quarks)
Notably, the d* hexaquark, consisting of three up and three down quarks, was discovered in 2014. While resembling the deuteron, it has a higher mass.
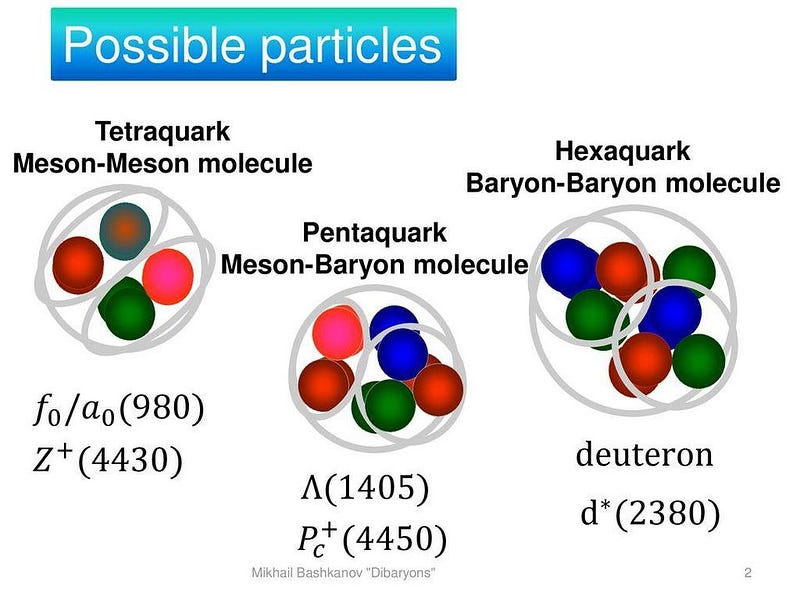
We have observed various particles like the rho mesons, which decay swiftly into lighter pions, and the delta baryons, which are also unstable. The d* hexaquark, an excited state of the deuteron, has a mass of 2380 MeV/c² and a similar decay time of 10^-23 seconds.
In contrast, dark matter must exhibit stability over billions of years and cannot decay on such short timescales. However, if sufficient d* hexaquarks formed in the early Universe, they could potentially bind together to create a stable state akin to a miniature neutron star.
A recent paper titled A new possibility for light-quark dark matter by M. Bashkanov and D.P. Watts explores this scenario, suggesting:
- Bound states of six quarks could behave as bosons.
- The d* may be smaller than a proton.
- In the dense conditions of the early Universe, numerous d* particles might condense into a Bose-Einstein state.
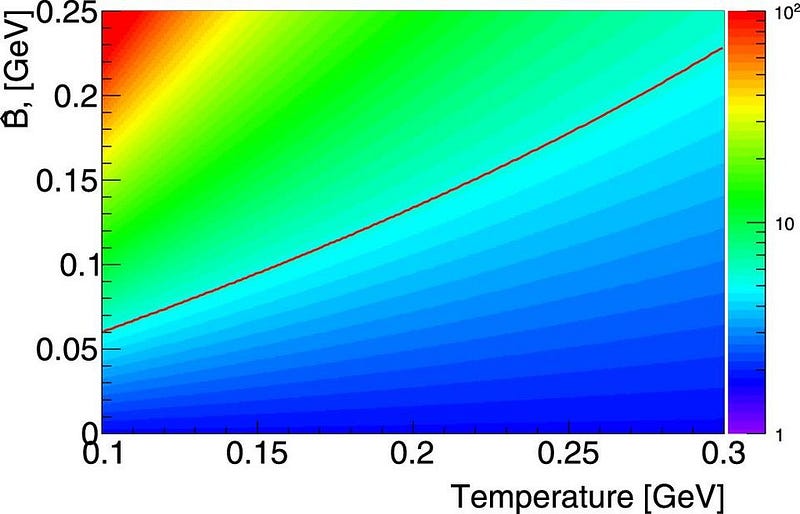
If these conditions hold true, and if the binding energy is substantial enough (around 10% of the d*'s rest mass), it may prevent the typical decay of the d* due to energy constraints, similar to how beta decay is inhibited in normal deuterons. This concept is certainly intriguing and could be explored in heavy ion collider experiments.
Nevertheless, even if the authors' claims are accurate — even if quarks separate and form significant numbers of d* particles shortly after the Big Bang — the survival of these d* particles is unlikely. The early Universe was predominantly radiation-dominated, with high-energy particles colliding frequently, which would likely disrupt any d* formations.
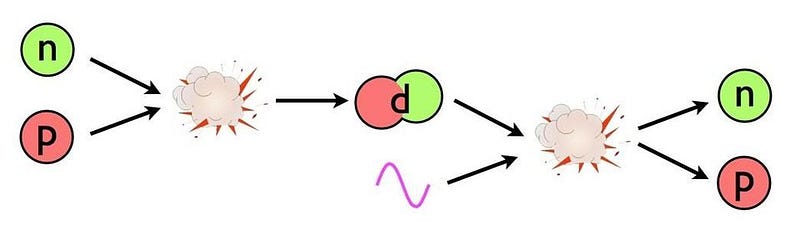
This challenge extends to all composite particles. It's the reason normal deuterium does not form until the Universe is about three minutes old; radiation continuously disrupts potential deuteron formation. For d* hexaquarks formed in the microsecond timeframe, the same issue arises, with no viable solution: radiation would prevent their stability.
To conclude, while it's essential to consider exotic states of matter and their potential stability, the reality of the early Universe imposes significant hurdles. We must ensure that we can produce these particles while avoiding their destruction. Based on our current understanding, this remains an elusive goal.
In summary, while the idea of hexaquarks as dark matter is innovative and avoids typical pitfalls, the intense radiation of the early Universe makes their survival improbable. Once disrupted, there would be no opportunity for d* particles to reconstitute and form Bose-Einstein condensates, as those creation conditions would have already passed. Thus, the early Universe, as we currently comprehend it, effectively challenges the feasibility of d* hexaquarks constituting dark matter.
Feel free to send your Ask Ethan queries to startswithabang at gmail dot com!
Starts With A Bang is featured on Forbes and republished on Medium with a one-week delay. Ethan has authored two books: Beyond The Galaxy and Treknology: The Science of Star Trek from Tricorders to Warp Drive.